Schizochroal eyes were big, but not because of the number of facets they contained, which were surprisingly sparse. Instead they owed their size to the vastness of each facet - up to a whole millimetre in diameter, a dimension not even approached in today's compound eyes. A boundary region separated each facet from its neighbours, and the lenses were either elongated prisms or came in two parts that locked together, one above the other.
The two-part lens is interesting. In addition to flying a kite into a thunderstorm in an attempt to understand electricity, the American diplomat and scientist Benjamin Franklin was also famous for inventing bifocal glasses in the eighteenth century. These offered the wearer the choice of seeing objects either near or far with accuracy. The trilobite schizochroal eyes with the two-part lenses did the same thing, so that they could see both tiny prey within grasping range and enemies approaching from a safe distance. The graded material lenses first identified by Maxwell in his breakfast herring are also to be found in some schizochroal eyes. Making a direct comparison, these trilobite eyes may have worked like those superposition eyes with graded lenses, such as are found in a group of crustaceans called mysids. On top of this, a new type of eye was found recently in a living insect called a strepsipteran, and this may unlock the secrets of the enormousness of the schizochroal facets.
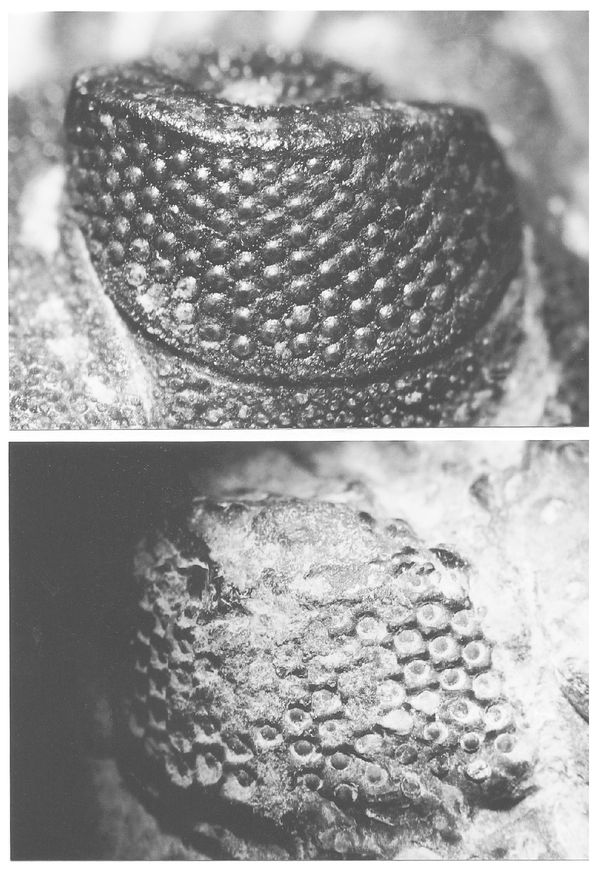
Figure 7.13
Photographs of holochroal (above) and schizochroal (below) trilobite eyes.
Strepsipterans are tiny insects that parasitise wasps. The eye of the male strepsipteran has only fifty lenses, as opposed to seven hundred in the similar sized
Drosophila
fruit flies, but they are each relatively huge. Each lens is linked to its own retina, and the nerves serving the individual retinas cross over, so that a complete picture can be assembled in the brain with everything in the right place. This form of imaging, which was probably echoed in the schizochroal trilobite eye, falls somewhere between that of compound and simple eyes.
Efficient as it may seem, and strepsipterans aside, the schizochroal eye was confined to just one group of trilobites known as the Phacopina. Phacopina lived up until 370 million years ago, but they did not evolve until the very end of the Cambrian period, around 510 million years ago. The schizochroal eye evolved
from
the trilobite eye of most interest in this chapter - the holochroal type. This has a significantly earlier origin.
Holochroal eyes generally contained more facets than schizochroal eyes, where each facet was relatively small. The lenses were simple - thin and biconvex (âoval'), like the lenses of magnifying glasses. They were packed together in a square or hexagonal formation, where neighbouring lenses were touching. But exactly how the holochroal eye functioned is something of a mystery. The real problem is that part of the eye may or may not be missing in the fossils. We do not know. The calcite lenses have preserved well thanks to their chemistry. But were there further focusing elements lying behind these that just haven't preserved because of an unfavourable chemistry for fossilisation? In one
way, taking a strict view of their position in the eye, the calcite lenses of trilobites could be more comparable to the thick corneas of modern compound eyes. In which case we
would
expect a further focusing element, or lens, to be lying just beneath. But then again, maybe the calcite lenses were the
only
focusing elements of holochroal eyes in trilobites, and maybe these were quite adequate.
So the internal architecture of the trilobite eye, as it is known, cannot provide the information needed to make comparisons with modern compound eyes. But there are some clues to be found on the outside. Those holochroal eyes with square-shaped facets may be comparable to the reflecting type of superposition compound eye, where the facets are square for a reason. These are the facets lined with mirrors, and the mirrors perform the focusing. And then those holochroal eyes with hexagonal-shaped facets may have worked like today's apposition compound eyes, with stark similarities on the outside. If these inferences are correct, we could predict the environments or lifestyles of the trilobites.
One shrimp today changes its eye throughout its development from juvenile to adult. As a juvenile it has an eye with hexagonal facets - an adaptation to its bright, shallow-water environment. This apposition eye is good at producing sharp images, but not so good at collecting all the light available. Fortunately there is a plentiful supply of light for this juvenile. But as it grows, it migrates to deeper waters, where light becomes more limited. So the apposition eye is shed during the moult to adulthood, and is replaced by a superposition eye with square, mirror-box lenses. This new eye has quite the opposite properties; although not effective at forming sharp images, it can make the most of the light available. All of this evidence suggests that trilobites with hexagonal-shaped facets lived in shallow waters, and those with square-shaped facets lived deeper, or were active at night.
On the other hand, square and hexagonal shaped corneas may be consequences of lens-packing geometry alone - the way they are squashed together. Because an alternative exists, unless completely preserved holochroal-type eyes are found in the future we will never know with certainty exactly how this organ worked and, consequently, how its hosts viewed the world. For the purposes of this book, it is enough to say with confidence that trilobite eyes produced visual
images - trilobites with these eyes could see. Now we should move on to more important matters.
Although the origin of the holochroal eye
now
appears to pose a big question, it is a question that has never been properly addressed. Without the line of enquiry maintained in this chapter, there is little justification for pursuing such an otherwise unimportant goal within the gravity of science. But in this chapter we have been deliberately building up to the very first eye in existence on Earth. Through a process of elimination we have arrived at the holochroal compound eye of trilobites. And from here we are in the hands of palaeontologists. We must rely on the fossils to help provide us with a date for that
very first
holochroal eye - the
very first eye
. The fossils do not let us down.
The oldest trilobites known are from the Lower Cambrian - the earliest part of the Cambrian. So far, so good. But we can be even more precise than that. The very first trilobites evolved at the
very beginning
of the Cambrian, around 543 million years ago - and they were equipped with holochroal compound eyes. Before this date there were neither trilobites nor eyes on Earth. So it is worth a look at those first trilobites and their eyes.
The oldest known,
well-preserved
trilobite eyes were described by Euan Clarkson, an expert on trilobite eyes at the University of Edinburgh, and his colleague Zhang Xi-guang from the Chengdu Institute of Geology in China. Working on material from south central China, they found particularly interesting compound eyes in two species of trilobite -
Neocobboldia chinlinica
and
Shizhudiscus longquanensis
.
Xi-guang and Clarkson used acid to dissolve the limestone slabs excavated from their Lower Cambrian site. The trilobites were freed from their matrix and were ready to be studied in electron microscopes. They had been particularly well preserved, thanks to the protection afforded by a phosphate coat, and so fine details of their optics could be uncovered.
Neocobboldia
bore a thick lens in each facet of its eye, and the lens was free from spherical aberration, the problem created when rays entering different parts of the lens are focused on to different planes. But it showed no signs of a graded material lens like that of the herring
or some compound eyes today. How was spherical aberration avoided? The answer lay in a sophisticated design, involving a precisely curved divide within the lens. This âintralensar bowl' design was not new to science - Huygens and Descartes had invented something similar in the seventeenth century - but here trilobites were proving that it really worked.
The lenses are less well preserved in the eyes of
Shizhudiscus
, although they are obviously simpler in design - they are biconvex (âoval'). All things considered, these eyes conform to the rules of the holochroal type and so they could produce visual images in the very Early Cambrian. And nice panoramic pictures, too - images of anything positioned on the trilobites' horizon.
Figure 7.14
The intralensar bowl design in the lenses of some trilobites; light rays striking all parts of the lens are focused in the same plane. An identically shaped lens without the intralensar bowl is shown for comparison.
In fact there are a number of trilobite species known from the very beginning of the Cambrian, and all have eyes, although they are not so well preserved. Even Charles Doolittle Walcott suspected this trend in 1910. And in 1957 the trilobite specialist Frank Raw, of Birmingham University in England, declared, âHow ancient already in the Lower Cambrian must the compound eye have been.' This statement was seconded by Euan Clarkson in 1973. Another case of trilobite eyes is that of
Fallotaspis
from Morocco, around 540 million years old.
Fallotaspis
had large eyes. The list continues . . .
Figure 7.15
Time ranges of genera within the seven families of trilobite, showing the occurrence of different kinds of eye (after Euan Clarkson, 1973). Note that the very first trilobites, living at the base of the Cambrian, bore (holochroal) eyes.
To reiterate, there is one curious but common fact emerging from the trilobite eye data. Many species of trilobites with eyes came into existence around 543 million years ago . . . but not a single species before that time. The trilobites
without
eyes entered history a little later, in geological time. So 543 million years ago the Earth witnessed the first trilobite . . . and the first eye. Five hundred and forty-three million could be a magic number.
An important question in this study is, âHow quickly can an eye evolve from its forebears?' Fossil evidence implies that eyes existed 543 million years ago but not before. Not, say, 544 million years ago. But surely an eye cannot simply evolve overnight? Surely it has to pass through a sequence of intermediate stages, probably within intermediate species in the evolutionary tree? These intermediate species must have fallen between the completely eyeless ancestor and the first eyed ascendants. If some of these intermediate species could see, albeit in a rudimentary way, and if they existed millions of years before the first ideal eye, then maybe animals acquired vision earlier than 543 million years ago. Maybe the introduction of eyes on Earth was staggered; perhaps sharp images were seen millions of years after blurred images, with visual precision increasing systematically. Dan-Eric Nilsson and his colleague at Lund University, Susanne Pelger, have deciphered a series of intermediate stages for the evolution of a camera-type eye. More than that, they have calculated the time needed to complete the sequence via the process of evolution. This is just the data we need.