Science fiction pulps often cited magnetism as the basis for a variety of technological wonders. Magnets were frequently called upon as a catch-all explanation for levitating heavier-than-air ships, while “reverse magnetism” was often invoked for force beams or other offensive weaponry. Readers of the daily newspaper’s comic-strip page have known since the mid-1960s that personal flying devices would someday be a reality, thanks to magnetism. Figure 8 shows a panel from a 1960s Dick Tracy comic strip, where Tracy, in silhouette, and his partner, Detective Sam Catchem, are able to scout for criminals using magnetized flying garbage cans. (Tracy is also carrying on a conversation using a “two-way wrist radio,” an early form of the cell phone.) Magnetism was expected to usher in the world of tomorrow—as the panel reproduced in Figure 8 promised, “The nation that controls magnetism will control the universe.”
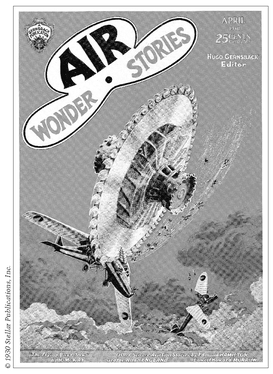
Figure 9:
Angular momentum was a frequently invoked physics principle for futuristic weapons of war, as shown in the cover of the April 1930 Air Wonder Stories.
Similarly, spinning is also a hallmark of the flying saucers and futuristic weapons (or sometimes both, as shown on the cover of the April 1930 issue of
Air Wonder Stories
in Figure 9). It would have to wait for the full relativistic form of quantum mechanics, developed by Paul Adrien Maurice Dirac in 1928, to show the fundamental connection between internal rotation and magnetism.
The third quantum principle states that everything, matter and photons, has an internal rotation about an axis that passes through the object, like a twirling figure skater. For ordinary matter, there is only one question about the rotation—clockwise or counterclockwise? In the previous chapter we discussed linear momentum defined as the product of an object’s mass and velocity. Since the objects in Chapter 3 were moving in straight lines, we could employ the linguistic shortcut and just refer to it as “momentum” rather than the more accurate term “linear momentum.” The greater an object’s momentum, the harder it is to change its motion. A baseball thrown at 100 miles per hour has more momentum than one thrown at 1 mile per hour; the latter may be arrested safely bare-handed, without a catcher’s mitt, while I wouldn’t recommend this method for the former (in fact, you’d need to stand pretty close to the pitcher in order to catch the slower ball before it fell to the ground).
Similarly, “angular momentum” is the rotational analog of “linear momentum.” The rotation may be about an axis passing through the object, as is seen in a spinning top, or about a distant axis, exemplified by the moon orbiting the Earth. In quantum physics, the spin of electrons or protons resembles a top or a ballerina more than it does an orbiting satellite. Moreover, the spinning of the particles within an atom is not arbitrary but
must
correspond to particular values of angular momentum. This is like saying that the linear momentum of a car can have two values, moving forward or backward at multiples of a given speed, such as 10 miles per hour. So the car could go 30 miles per hour forward or 30 miles per hour backward, but not, say, 13 miles per hour in either direction.
It turns out, based on experimental observation, that certain fundamental particles in the universe have an internal angular momentum that has a value of either 0 (a very special case) or Planck’s constant,
h,
divided by 2π. Photons, for example, have an intrinsic angular momentum of
h
/2π. Other fundamental particles, such as electrons, protons, and neutrons, can have an internal angular momentum of exactly one-half of this value of Planck’s constant,
h,
divided by 2π, that is, (1/2) × (
h
/2π). That’s it. Whether an object has an internal angular momentum that is either an integer multiplied by
h
/2π or a half-integer multiplied by
h
/2π will have a
profound
effect on how it interacts with other identical particles.
As 2π is just a number, if
h
/2π is a measure of angular momentum then Planck’s constant,
h,
is a unit of angular momentum. When Planck introduced the constant
h
as a fudge to account for the spectrum of light emitted by hot, glowing objects, he had hit upon a fundamental constant of the universe. There is a set of basic numbers that one must specify when setting up a universe, such as the mass of the electron and the speed of light. Things would look very different if the speed of light, for example, was a value a person could achieve while riding a bicycle, such as 15 miles per hour. One would then have an intimate, firsthand intuition about the consequences of the Special Theory of Relativity. Similarly, if Planck’s constant were a much larger number, we would have to deal with quantum phenomena in our daily lives.
In Isaac Asimov’s novel
Fantastic Voyage II: Destination Brain,
a team of scientists is reduced in size, smaller than a single cell, in order to travel within the body of an injured scientist (who has figured out a way to make miniaturization energy efficient!) and perform an operation. Asimov proposes that the mechanism underlying this shrinking process involves creating a field that reduces the magnitude of Planck’s constant. Considering an atom to be a sphere, Bohr calculated its radius to be a few times r
o
, where r
o
=
h
/ [(2π)m
e
c α] and m
e
is the mass of the electron, c is the speed of light, and α is termed “the fine structure constant” that involves another collection of fundamental constants (such as
h,
c, and the charge of the electron). If one could tune Planck’s constant at will, making it larger or smaller, then one could enlarge or shrink any object by changing the fundamental size of its atoms.
16
The fact that we cannot do this in reality reflects the fact that fundamental constants are just that—constant and unchanging.
It was unnerving to physicists when Albert Einstein suggested in 1905 that there was no velocity faster than light speed, but the universe and its laws indeed ensure that nothing can move faster than the speed of light in a vacuum. Apparently, with the discovery that subatomic particles have internal angular momentums whose values are multiples of either
h
/2π or 1/2 of
h
/2π but not any other values, the universe also cares about rotation.
The electron is the basic unit of negative charge, while the proton has an equal charge, but of an opposite sign (by convention the proton’s charge is termed “positive” while the electron’s is “negative”). It has been known since the 1820s that moving electric charges, that is, an electrical current, create a magnetic field. This is the basic physical principle underlying electromagnets and motors. If an electrically charged sphere rotates about a line passing through its center, like a wheel about its center spoke, then there are certainly electrical charges in motion, and these currents will generate a magnetic field. If there is an intrinsic angular momentum, it shouldn’t be surprising that as a consequence of this rotation every electron and proton has its own internal magnetic field. In fact, the quantized internal angular momentum aspect was proposed to account for the experimentally observed internal magnetic fields inside atoms. That is, the observation of the magnetic field came first, and later, in an attempt to account for it, the argument about intrinsic angular momentum was put forward.
Does the experimentally observed magnetic field of electrons and protons actually arise from the spinning rotation of elementary particles? Technically, the answer is no. The simplest reason why not is that neutrons, the other fundamental particle found within atomic nuclei, which have nearly the same mass as protons but are electrically uncharged,
also
possesses an internal magnetic field! If the magnetic field of the proton arose from the fact that as a charged object, its rotation could be described as a series of electrical current loops, each of which generates a magnetic field, then the rotation of an electrically uncharged object should not generate a magnetic field.
17
Moreover, even if we did not know that neutrons existed, we still could not explain the magnetic field of electrons as arising from their rotation about an axis passing through their center. The magnitude of the measured magnetic field of the electron is such that it would require that these particles spin at a rate so fast that points on their surface would be moving faster than the speed of light!
What experimental question does the proposal of intrinsic angular momentum (that is, spin) answer? In 1922 Otto Stern and Walther Gerlach passed beams of atoms through special magnets, looking for interactions between their laboratory magnet and the internal magnetic field of the atom. They were trying to probe the magnetic field that would arise from the electron’s orbital motion about the nucleus. For atomic systems where they did not expect to see any orbital motion, they nevertheless observed an intrinsic magnetic field of elementary particles and, moreover, that this came in two values. It was if the electron had a built-in magnetic field with a north pole and a south pole that was allowed to point in only two directions, relative to the magnets used in Stern and Gerlach’s experiment. The electron either pointed in the same direction as the external magnet, so that its north pole faced the south pole of the lab magnet, or exactly oppositely aligned, so that the electron’s north pole faced the lab magnet’s north pole.
While Stern and Gerlach’s experiment clearly suggested that electrons possessed an intrinsic magnetic field, spin was actually first proposed to account for features in the absorption and emission of light by certain elements that indicated that there had to be some internal magnetism inside the atom. A variety of careful experiments confirmed that this magnetic field did not arise from the electrons orbiting around the positively charged nucleus, but was somehow coming from the electrons themselves.
So, why do we say that electrons, protons, and neutrons have spin that is associated with their internal magnetic fields? The origins of this phrase go back to, shall we say, a “youthful indiscretion.” Two Dutch graduate students in Leiden, Samuel Goudsmit and George Uhlenbeck, wrote a paper in 1925 suggesting that an internal rotation of the charged electron generated a magnetic field necessary to account for the atomic light-emission-spectra anomalies. They showed their paper to their physics adviser, Paul Ehrenfest, who pointed out various problems with the electron literally spinning about an internal axis. Hendrik Lorentz, Ehrenfest’s predecessor, soon calculated, as the students’ argument required the electron to rotate faster than light speed, that, thanks to E = mc
2
, this would make the electron heavier than the proton. (Neutrons, which would have indicated to them immediately that the observed magnetic field could not result from a spinning charged particle, had not yet been discovered.) Defeated, Goudsmit and Uhlenbeck intended to drop the whole matter. They were surprised when Ehrenfest told them that that he had already submitted their paper for publication. He consoled them, indicating that he had recognized their error but thought that there was merit in their suggestion, and argued that they were “young enough to be able to afford a stupidity.”
On one level it is unfortunate that Goudsmit and Uhlenbeck employed the term “spin” for the intrinsic angular momentum and magnetic field possessed by subatomic particles. The term is so evocative (and the fact that an electron, for example, can have an intrinsic angular momentum of either +(1/2)
h
/2π or -(1/2)
h
/2π, but no other values, makes it easy to think of in terms of “clockwise” or “counterclockwise” rotation) that it is difficult to remember that the electron is not actually spinning like a top. The intrinsic angular momentum of the electron is properly accounted for in the Dirac equation, a fully relativistic version of quantum theory. Solving the Dirac equation, one finds that the electron is characterized by an extra “quantum number” that corresponds to an internal angular momentum of (1/2)
h
/2π and a magnetic field of magnitude exactly as observed. In a sense it is an intrinsic feature of the electron, just like its mass and its electric charge.
18
Goudsmit and Uhlenbeck managed to get the right answer for the wrong reasons. For this work they were awarded several elite prizes and medals. Publish in haste, celebrate at leisure.
I have promised that this internal angular momentum, possessed by electrons, protons, neutrons, and all other elementary particles, is the key to understanding the periodic table of the elements, chemistry, and solid-state physics. In Chapter 12 I will describe the Pauli exclusion principle, which states that when two electrons (or two protons or two neutrons) are so close to each other that their de Broglie waves overlap, they can both be in the same quantum state only if one electron has a spin of
+h
/2π and the other has a spin of −
h
/2π. This has the consequence of “hiding,” except in certain cases, the magnetism associated with the intrinsic angular momentum.