Figure 26:
A sketch of a 1936 Buck Rogers toy ray gun and nuclear radioactivity consisting of alpha particles, beta rays, and gamma ray photons. (The toy shown is a Buck Rogers Disintegrator Pistol, model XZ-38, and did not actually emit high-energy sub-atomic particles). All three types of radiation have roughly the same energy of a few million electron Volts, but they are stopped by different levels of shielding. The alphas are blocked by a sheet of paper, which the betas can penetrate, but are themselves stopped by a thin aluminum sheet. Highenergy light in the form of gamma rays passes through both, and a thick block of lead is needed to stop them.
Gamma-ray photons are high-energy light, several hundred times more energetic than X-rays. As gamma rays are photons of light and uncharged, they do not interact directly with the electronic charges in atoms, which makes them much harder to stop. It takes about one-half inch to an inch of dense material such as lead to stop gammas, and they can penetrate through an entire person. Some nuclei (such as uranium 238) may also emit neutrons
42
that in themselves are not harmful, but when they collide with hydrogen atoms in the body, the resulting high-speed ricocheting protons can be damaging.
If you are unfortunate enough to ingest an unstable nucleus, so that it is inside you when it undergoes radioactive decay, then even alpha particles can be deadly. Rather than striking the dead skin cells on your epidermis, which you slough off naturally, alpha particles inside you have a direct path to your internal organs. In this case the alpha particles prove to be very efficient in stripping electrons from the atoms they strike, disrupting the chemical bonds within the cell and causing extensive chromosomal damage.
In 2006 Russian journalist Alexander Litvinenko was murdered when he drank tea that was spiked with polonium 210. This unstable nucleus has a half-life of just over 138 days and emits high-energy alpha particles when it decays. A pound of polonium 210 releases energy at the rate of nearly 64,000 Watts. Because its probability of radioactive decay is so high, even 0.05 micrograms of polonium 210 is considered to be lethal (it is believed that Litvinenko had 10 micrograms in his body at the time of death). So, alphas on the outside, not too much of a problem—on the inside, a rather big problem. Which is probably why it was fortunate that Gilbert stopped marketing their U-238 Atomic Energy Lab in 1952. I mentioned in the last chapter that this kit contained a mini- cloud chamber. Part of the radioactive elements supplied with this chamber was a small piece of potent, though short-lived, radioactive polonium 210!
Of course, the fact that some forms of radiation can cause sufficient damage to kill the living cells they impinge can be a good thing. The cells in a piece of meat from the butcher are nonliving, but the bacteria within the meat that can cause salmonella or other diseases are very much alive. Exposure to radiation does no significant damage to the cells in the food that are already dead but can penetrate and kill the bacteria living in the food, thus making the food much safer to eat. The exposure to radiation does not convert the stable nuclei in the food’s atoms into unstable nuclei, and they will not in turn emit their own radioactivity. A material that has been irradiated does not (with very few exceptions) itself become radioactive.
A lot of the harm of nuclear radiation is caused when either negatively charged beta rays or positively charged alphas or gamma-ray photons collide with atoms and cause them to lose their electrons. This process is called “ionization,” and when an atom loses some or all of its electrons, its chemical properties can be radically changed. Sometimes these changes are beneficial. The Earth is constantly being bombarded with cosmic rays, which are primarily (though not exclusively) high-energy protons that come from sources as close as our sun and as distant as other galaxies. When these protons (a few of which have energies of a million trillion electron Volts) strike the atmosphere, they can generate a slew of other elementary particles moving near the speed of light. When some of these particles strike the DNA in our cells, they can cause ionizing damage and alter the chemical properties of our genetic code. If the affected DNA is in a sperm or egg cell, these changes may be passed along to offspring. In this way exposure to cosmic rays is a natural source of genetic mutation, leading to biological modifications that can be harmful but occasionally improve an organism’s fitness to its environment.
But beneficial mutations that do not harm the original organism and lead to genetic alterations that improve the offspring’s reproductive success are extremely rare. More commonly, chemical modifications induced by ionizing radiation can destroy cells themselves or induce deleterious alterations in chromosomes or DNA. This damage often leads to the formation of malignant cancerous tumors, quite different from the runaway cell growth presented in science fiction movies such as
The Amazing Colossal Man
or
The Attack of the Giant Leeches.
CHAPTER ELEVEN
Man of the Atom
Before there was physicist
Jon Osterman, there was physicist Philip Solar. In the 1986 DC comic book
Watchmen,
Osterman was disintegrated by the accidental removal of his intrinsic field at Gila Flats and reconstructed himself as the superpowered Dr. Manhattan. In the 1962 Gold Key comic book series
Solar—Man of the Atom,
Phillip Solar was exposed to a lethal dose of radiation in a sabotaged nuclear research experiment at Atom City, yet survived, though he acquired “quantum powers.” In issue # 2 he is vaporized by an atomic bomb blast but manages through sheer force of will to reconstitute himself as, well, Dr. Solar, which was his name after all. As a survivor of graduate school myself, I can empathize with Osterman and Solar’s inclination to retain the title associated with their Ph.D.’s, in the lab or as a superpowered hero. Once you’ve passed through the crucible of a graduate school candidacy exam, having to reassemble yourself up from the subatomic level is not as challenging as you might think.
In writer Alan Moore’s initial outline of the DC comic book miniseries
Watchmen,
he intended to use comic book characters created by Charlton, another comic book publisher. Charlton had declared bankruptcy, and the company had been acquired by DC Comics, home of Superman and Batman. Moore’s initial outline for
Watchmen
made direct use of the Charlton characters, but the editors at DC Comics, seeing that some of these characters would not make it out of the miniseries unscathed, instructed Moore to 8 instead employ alternate versions of the Charlton heroes. Dr. Manhattan is the analog of Captain Atom, an air force captain, who was disintegrated and (I’m sure you can see this coming at this stage) through force of will was able reassemble himself into a quantum-powered superbeing.
Captain Atom’s powers were quantum based only in that he was able to manipulate energy, which he employed primarily for flight, superstrength, and energy blasts. Dr. Solar, though not a Charlton character, seems to be a closer antecedent for Dr. Manhattan, as Solar was also able to change size (
Dr. Solar
# 10 and # 11), split himself into multiple copies of himself (
Dr. Solar
# 12), and manipulate matter and energy, though unlike the blue Dr. Manhattan, Dr. Solar’s skin turned green when he used his powers. There are just enough differences among Captain Atom, Dr. Solar, and Dr. Manhattan that it is unlikely that they are all the same person, on three different versions of Hugh Everett’s many worlds, though further study appears warranted.
One of the more accurate manifestations of quantum mechanical powers was presented in “Solar’s Midas Touch,” in 1965’s
Dr. Solar, Man of the Atom
# 14. In this tale an underwater nuclear reactor pile went critical when one of the control rods (whose role is to absorb neutrons, decreasing the rate of uranium fission, as described in Chapter 9) broke. Dr. Solar, whose powers are normally energized by exposure to radiation, went underwater to fix the reactor but found himself weakened by the reactor’s radioactivity (through a process not clearly explained in the comic). Eventually he was rescued by a worker wearing a lead-lined safety suit, who would have done the job in the first place if Dr. Solar hadn’t attempted to “save the day.” The additional radiation he absorbed from the reactor temporarily endowed Solar with a new superpower. As illustrated in Figure 27, whenever Dr. Solar comes into physical contact with an object, he transmutes it into the next element up the periodic table. In Figure 27, he transforms gold, with seventy-nine protons, into mercury, with eighty protons; earlier he grasps a copper rod (twenty-nine protons) and converts it into zinc (atomic number 30); and even when flying he begins to choke when the oxygen (atomic number 8) turns into fluorine gas (with nine protons). This newfound power of Dr. Solar’s appears to be the abil-ity to initiate beta decay of the neutrons in any object he touches, inducing elemental transmutation via the weak nuclear force, an aspect of Watchmen’s “intrinsic field” that we have not discussed much yet.
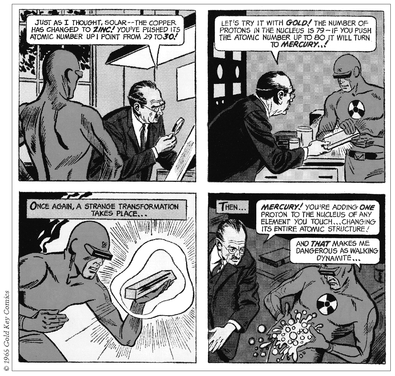
Figure 27:
In
Dr. Solar, Man of the Atom # 14
, an additional nuclear accident endows Dr. Philip Solar (wearing the scuba suit and visor) with the temporary ability to induce beta decay via the weak nuclear force in any object he comes into direct contact with, thus transmuting gold into mercury.
We saw in Chapter 9 that neutrons, through the strong force, hold the nucleus together by binding with protons and other neutrons and overwhelming the electrostatic repulsion that would, in their absence, cause the protons to fly out of the nucleus. Protons also exhibit the strong force, but without neutrons there is not sufficient binding energy to hold together a nucleus consisting only of protons. Neutrons themselves are not stable outside of a nucleus. A neutron sitting alone in the lab will decay into a proton and an electron with a half-life of about ten and one quarter minutes. The electron will be moving very near the speed of light, and when this process occurs within a nucleus, it is the source of the beta rays emitted from unstable isotopes.
As the total mass and energy of an isolated system must remain unchanged in any process, a “stationary” neutron
43
can decay only to fundamental particles with less mass than the neutron’s. A neutron will thus decay into a proton, which has a slightly smaller mass, while a “stationary” proton could not decay into a heavier neutron. However, as the neutron is electrically neutral, and the proton is positively charged, the decay must also generate a negatively charged electron, in order for the total electrical charge to remain unchanged before and after the decay (we have not needed to invoke this principle before now, but another conservation principle in physics, comparable to conservation of energy or conservation of angular momentum, is conservation of charge, in that the net electrical charge can be neither created nor destroyed in any process). An electron is nearly two thousand times lighter than a proton, less than the mass difference between neutrons and protons, so adding an electron to the decay is still consistent with mass conservation. While a neutron decaying into a proton and an electron means that mass and electrical charge are balanced during the decay, examination of the kinetic energy of the proton and the high-speed electron (that is, the beta ray) and comparison to the rest-mass energy of the neutron indicates that some energy went missing in the process—not a lot, but enough to notice, and enough to cause trouble.
When physicists in the late 1920s discovered this phenomenon and realized that it appeared to violate the principle of conservation of energy, they were faced with two choices: (1) either abandon conservation of energy, at least for neutron decay processes, or (2) invent a miracle particle that was undetectable by instrumentation of the time but that carried off the missing energy. In 1930, Wolfgang Pauli (whose exclusion principle I address in the next section) suggested going with option 2. Knowing that this ghost particle had to be electrically neutral and had to have very little or no mass, Enrico Fermi called it the “little neutral one” in Italian, or “neutrino.”
44